Article based on original text published in the author’s doctoral dissertation on July 10, 2020.
The number of literary works published to this day about the Moon and its environment abounds. From lunar formation theories to the study of the chemical composition of its crust and the provenance of its topography, plenty are the options awaiting the avid reader. New data collected by current lunar orbiters improves our ever-changing understanding of the Moon, its origin and evolution, refuting some previous theories and providing evidence for new ones.
In this brief article, I will provide you with key information about our neighboring celestial body. This information has been gathered from multiple sources, including books, papers, and news articles, which you can find listed at the end. By the end, you will be able to understand broadly the reasons that make the Moon such an awe-inspiring, mysterious, and fundamental target for visionaries, explorers, scientists, technologists, and entrepreneurs alike. This article won’t solve all your questions, some of which scientists may not even have the answers to yet, but should help you define a starting point in your quest to discover what’s yet to explore.
Welcome to the Moon
With a surface gravity of 1.622 m/s2 and a diameter of 3474 km, the Moon is the fifth largest satellite in the Solar System after Ganymede, Titan, Calisto, and IO. Its surface extends over an area of 37.9 million km2, 7.5% that of Earth and 26% that of Mars.
The Moon orbits Earth in a prograde orbit, meaning it travels in the same direction as Earth’s rotation. The Moon has a sidereal period of 27.3 days—its orbital period around Earth—and a synodic period—from new moon to new moon—of 29.5 days. This shift is due to the constant movement of the Earth-Moon system around the Sun, which in turn gives rise to the Moon phases we experience on Earth.
Being tidally locked to Earth, the lunar farside is naturally isolated from Earth’s ionosphere and electromagnetic interference, which is why this particular location on the Moon is thought to be the perfect and quietest spot for astronomical investigations to take place [1]. Astrophysicists and cosmologists believe missions operating on the far side of the Moon would have access to the information needed to address some of the most profound questions regarding the early days of the universe formation [2].
Topography
The Moon has a complex and extreme topography, with a maximum elevation point 1.9 km higher than Mount Everest and basins as deep as 9 km. The lunar topography is mainly characterized by an outermost layer of regolith—a fine, sharp, and electrostatically charged soil that covers most part of the underlying bedrock—and craters whose sizes range from micrometers to that of the South Pole-Aitken basin of about 2500 km in diameter—the oldest basin on the Moon and one of the largest known in the Solar System.
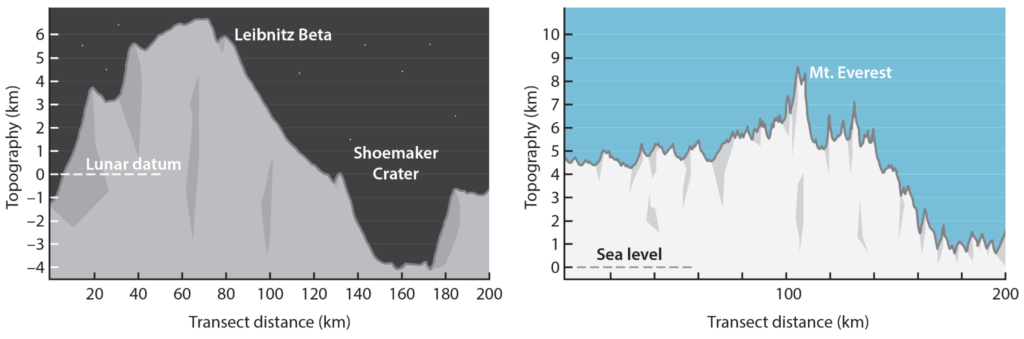
A transect of the summit of the Leibnitz β (Beta) massif including the Shoemaker crater, both located in the South Pole-Aitken basin, depicted alongside the elevation of Earth’s Mount Everest above sea level. The change in elevation at Leibnitz β exceeds 10 km, the highest elevation in the region. Credit: LPI/CLSE
The morphology of lunar craters has been influenced by impacts, erosion, and degradation processes [3]. If we were to macroscopically divide the lunar surface, it would be broken up into two main regions: the highlands and the maria, from the Latin “seas.”
The highlands are bright old regions thought to represent the original crust of the Moon. They are predominantly characterized by anorthositic rocks, those containing a high percentage (over 90%) of calcium-rich minerals.
The lunar maria, on the other hand, are dark basins characterized by basaltic lava flows, volcanic domes, rilles, and pyroclastic deposits. The latter are considered to be one of the predominant locations in the search for samples and potential resources since, scientists believe, pyroclastic deposits are the result of early lunar volcanic processes [4]. Pyroclastic vents are essentially deposits of airborne ash ejected from volcanic eruptions, which may provide direct information from the mantle uplift located more than 200 km below the lunar surface.
While the highlands are rich in calcium, aluminum, silicon, and oxygen, mare basalts are richer in magnesium, iron, and titanium. For an in-depth description of lunar geology, its chemistry and physical nature, I’d recommend reading the extensive review made in [5].
Temperatures
Extreme temperature fluctuations also characterized the lunar environment. Temperature differences of about 280 degrees were measured during the Apollo missions to the lunar surface [1]. Maximum temperatures of 384K (101ºC) were registered at the Apollo 17 landing site [6]. Whereas global temperature measurements provided by the Lunar Reconnaissance Orbiter (LRO) Diviner Lunar Radiometer Experiment revealed temperatures as low as 20K (-253ºC) within permanently shadowed areas in the lunar South Pole [7].
Atmosphere & radiation
As is the case with Mercury and most moons in the Solar System, the lunar atmosphere is negligible compared to that of Earth [8]. A tenuous layer of particles of helium, neon, argon, and hydrogen was detected by Apollo 17 Lunar Atmospheric Composition Experiment (LACE) and has been recently measured by the Lyman Alpha Mapping Project (LAMP) instrument, a UV spectrograph aboard LRO [9] [10][11]. These particles derive primarily from outgassing and sputtering effects produced by solar radiation, cosmic rays, and meteoric impacts.
This layer of particles is so weakly bonded that in practical terms the Moon is considered to be subjected to a vacuum. The lack of an atmosphere and a magnetic field leaves the Moon without natural protection from ionizing radiation.
The lunar surface is constantly exposed to solar wind, solar energetic particles, and cosmic rays, which add to the list of hazards confronting missions to the lunar surface. To this list, we need to add a 2.5-second round-trip signal delay to Earth and the detrimental effects of dust and poor visibility, which, as stated by Apollo astronauts, was one of the main difficulties in dealing with and traversing over the lunar surface.
Despite all the aforementioned aspects, and in line with the boldness that so often characterizes human nature, multiple reasons support the selection of the Moon as a key stepping stone toward accomplishing a permanent human presence beyond Earth’s lowermost orbit.
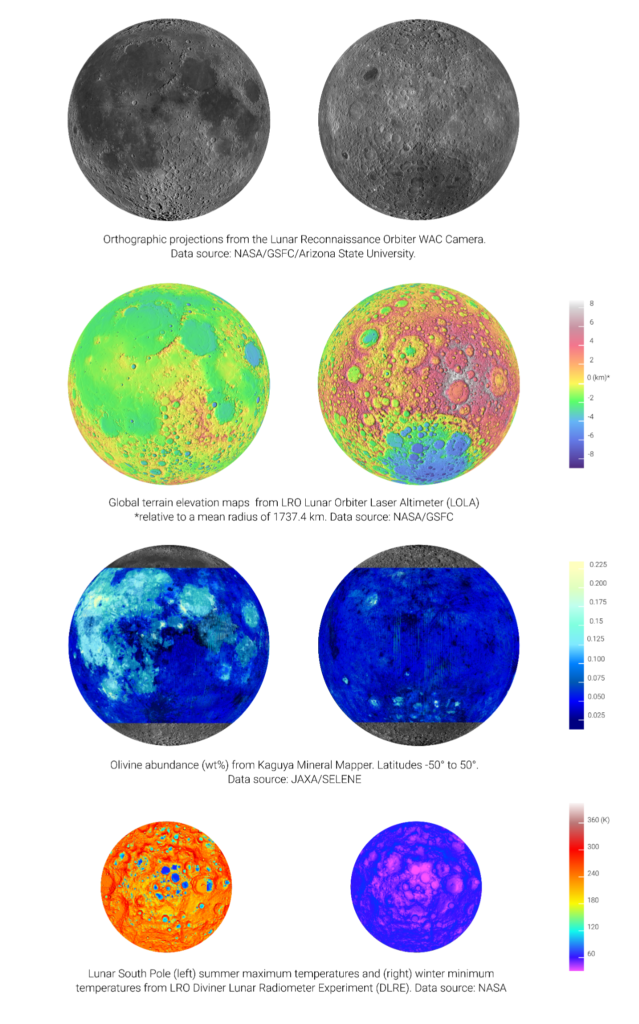
Visual representation of (left) near-side and (right) far-side lunar data. Images taken using LROC Quickmap Visualization Tool.
The potential of the Moon: lunar resources
Its proximity to Earth makes the Moon and cislunar space a perfect stop on the road to the almost infinite amount of resources resting in space. Extracting, processing, and using these resources is being referred to by some authors as the third great economic revolution: the space revolution [12].
The progressive increase in the world’s population and the rising standards of human welfare have drawn attention to the potential risk of depletion of Earth’s natural resources. Even though the consumption of certain resources has plateaued over time, others are being utilized at an incremental rate baring unsustainable in the long term, or even in the short term.
In an article published by D. Cohen in 2007 [13], several studies are cited in which it is estimated metals like platinum, silver, indium, or hafnium were just ten to fifteen years away from their complete depletion. Even if some may find these numbers to be overstated or based on misleading assumptions—they overlook in some cases the extraction of some of these elements as byproducts—, the truth is that Earth needs to be looked after [14]. The harmful consequences of the incremental use of fossil fuels and the potential scarcity of Earth’s natural resources have led scientists and decision-makers to consider how it would be possible to preserve actual human wealth. In [15] the author analyzes the different sets of potential resources that could be found on the Moon. In this article, the author highlights eight different types of lunar resources: solar wind implanted volatiles, helium-3, water, oxygen, metals, silicon, rare earth elements, and thorium and uranium. These can be used not just to support life on Earth but to expand it beyond the Kármán line.
Solar wind implanted volatiles
The lunar soil is constantly being bombarded by solar wind particles. These particles are mainly composed of hydrogen and, in less quantity, helium, which together with other implanted volatiles present in the regolith, such as carbon, nitrogen, fluorine, and chlorine, represent part of the full range of necessary life-sustaining consumables [1].
Helium
Helium, and in particular its scarce isotope helium-3, is considered an incredible source of energy. When fused with deuterium it produces an aneutronic reaction—a reaction in which no neutrons are released, which means that no radioactive waste is produced—that releases almost twenty times more energy per nucleon reaction than traditional fission processes.
On paper, the extraction and utilization of helium-3 as a potential source of energy seems to solve most of the problems of energy consumption faced in space or even on Earth. The spatial distribution and abundance of helium-3 in the lunar regolith are, however, yet to be characterized. And even if the abundance of helium-3 is such that its extraction becomes economically viable, technical difficulties inherent to the utilization of a deuterium-helium-3 fusion reactor would need to be considered in the first place [16].
Metals
Metals are an essential feedstock required to build up the infrastructure that supports scientific, exploratory, and industrial operations in space. Besides propellant, structural elements comprised the largest ballast of any space missions when attempting to overcome Earth’s gravity well. The most valuable elements to consider would be aluminum, titanium, iron, and other siderophile elements such as cobalt and nickel.
Iron is abundant in the lunar surface either in the form of basaltic rocks, native in the regolith, or localized in metallic meteorites shattered throughout the lunar surface. Since metallic meteorites are considered to have a modest presence of titanium, ilmenite-bearing rocks and other basaltic rocks (greater than 6 wt% of Ti) located throughout the lunar maria could become the primary source of titanium in the inner Solar System [17]. A similar case is that of aluminum. With a high presence in anorthositic rocks throughout the lunar highlands (10-18 wt%), aluminum is an element of minimum trace in both iron and stony Asteroids.
On the other hand, precious metals, such as gold and platinum group metals, which can also be found in space, are valuable on Earth but have little to no applicability in space [28].
Silicon
Another tremendously important element comprising one of the main constituents of solar cells is silicon. The anorthositic character of the Moon’s crust makes it rich in this valuable chemical element (about 20 wt% of lunar regolith is silicon).
Water
Despite the value of metals and semiconductors, the most sought-after of space resources is water. Due to its multiple uses, from the production of propellant and breathable oxygen for astronauts to drinking water and radiation shielding of high energy particles, water is driving the selection of prospective areas of exploration for governmental agencies and private corporations alike in the upcoming years.
Since the 1960s water in the form of ice has been suspected to be widely located in lower temperature regions in the lunar poles [19]. Due to the small inclination of the Moon’s equator to the ecliptic (1.5°), these regions are barely lit by sunlight or even remain permanently in shadow, acting as “cold traps” for volatile compounds.
Early on, the actual existence of water ice on the surface of the Moon had been in constant discussion within the scientific community. Observations made by Earth-based telescopes of regions expected to harbor high concentrations of exposed water ice were initially characterized by the lack of coherent backscatter [20]—a phenomenon produced by unusual radar echoes associated with the presence of thick deposits of ice similar to those observed in permanently shadowed regions of Mercury and Ceres [21][22]. The absence of this phenomenon was also confirmed by different observations made by the Selenological and Engineering Explorer (SELENE) spacecraft, a Japanese lunar orbiter dubbed Kaguya. Images taken by the SELENE Terrain Camera of the interior of Shackleton Crater—a crater located near the lunar South Pole and one of the leading candidates to host volatile compounds—confirmed the absence of exposed water ice in its central uplifts, an achievement that has been impossible to accomplish by Earth-based radar imaging techniques [23]. This claim was quite a setback in the expectation of water being ever found on the surface of the Moon.
Most recent observations and measurements made by the LCROSS and LRO missions of the Cabeus crater and south polar regions indicated the presence of highly dispersed, thin layers of surface and subsurface volatiles [24][25]. Polar subsurface volatiles mixed within the regolith grains were reported by the LRO Diviner instrument to be located not just within regions of permanent darkness but in some specific locations around the South Pole where sunlight strikes for just a few days every sidereal month [25].
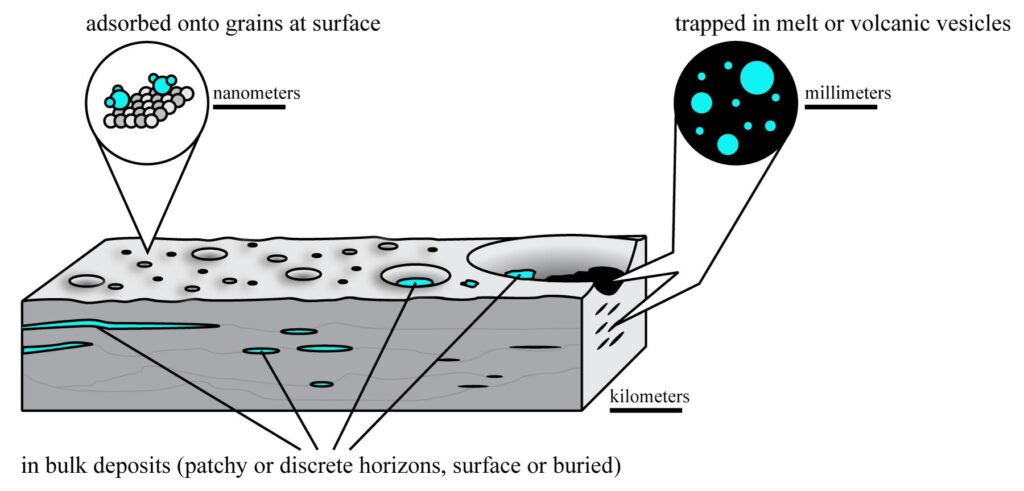
A simplified schematic of the potential location of lunar volatiles. Credit: LPI/CLSE
As a result of these findings, and others that are constantly being published by scientific teams all over the world, the lunar South Pole is being considered a prominent destination for future exploration missions.
A detailed characterization of the state, abundance, and distribution of water and other lunar resources is still a must for missions planned to launch within this decade. The challenging topography and reduced illumination of most of these areas may prove the characterization and extraction of these resources highly demanding in terms of energy, costs, and complexity. The scientific value of these compounds is undeniable. But whether the required resources are present in enough quantity to make the extraction worth the cost of exploitation is still an open debate.
Humankind has the responsibility of keeping curiosity, drive, and the spirit of exploration alive. They are parts of what make us who we are. Answers are needed to some profound questions and solutions are required for some vital problems. It is time we send our bots and our boots to the surface of the Moon. To seek the answers we need, to craft the solutions we demand, to explore, and this time to settle so that the human spirit never fades.
References:
- G. H. Heiken, D. T. Vaniman, and B. M. French. Lunar Sourcebook: a user’s guide to the Moon. Cambridge University Press, New York, NY, 1991.
- J. O. Burns, J. Lazio, S. Bale, J. Bowman, R. Bradley, C. Carilli, S. Furlanetto, G. Harker, A. Loeb, and J. Pritchard. Probing the first stars and black holes in the early Universe with the Dark Ages Radio Explorer (DARE). Advances in Space Research (in press), 2011.
- H. Hiesinger and J. W. Head Iii. New Views of Lunar Geoscience: An Introduction and Overview. Reviews in Mineralogy & Geochemistry, 60:1–81, 2006.
- S. Li and R. E. Milliken. Quantitative mapping of hydration in lunar pyroclastic deposits: Insights into water from the lunar interior. In the 45th Lunar and Planetary Science Conference (LPSC ’14), 2014.
- R. Jaumann, H. Hiesinger, M. Anand, I. Crawford, R. Wagner, F. Sohl, B. Jolliff, F. Scholten, M. Knapmeyer, H. Hoffmann, H. Hussmann, M. Grott, S. Hempel, U. K¨ohler, K. Krohn, N. Schmitz, J. Carpenter, M. Wieczorek, T. Spohn, M. Robinson, and J. Oberst. Geology, geochemistry, and geophysics of the Moon: Status of current understanding. Planetary and Space Science, 74(1):15–41, 2012.
- S. Keihm and M. J. Langseth. Surface brightness temperatures at the Apollo 17 heat flow site: Thermal conductivity of the upper 15 cm of regolith. In Proceedings of the Lunar Science Conference, vol 3, pages 2502–2513, 1973.
- J. Keller, N. Petro, and R. Vondrak. The Lunar Reconnaissance Orbiter Mission – Six years of science and exploration at the Moon. Icarus, 273:2–24, 2016
- S. A. Stern. The lunar atmosphere: History, status, current problems, and context. Reviews of Geophysics, 37(4):453–491, 1999.
- J. H. Hoffman, J. Hodges, R. R., F. S. Johnson, and D. E. Evans. Lunar atmospheric composition results from Apollo 17. In Lunar Science Conference, vol. 4, pages 2865–2875, 1973.
- S. A. Stern, K. D. Retherford, C. C. C. Tsang, P. D. Feldman, W. Pryor, and G. R. Gladstone. Lunar atmospheric helium detections by the LAMP UV spectrograph on the Lunar Reconnaissance Orbiter. Geophysical Research Letters, 39(12):1–4, 2012.
- S. A. Stern, J. C. Cook, J. Y. Chaufray, P. D. Feldman, G. R. Gladstone, and K. D. Retherford. Lunar atmospheric H2 detections by the LAMP UV spectrograph on the Lunar Reconnaissance Orbiter. Icarus, 226(2):1210–1213, 2013.
- G. Sowers. Creating a viable cislunar economy. ROOM: The Space Journal2, 4(14): 22–26, 2018.
- D. Cohen. Earth’s natural wealth: an audit. New Scientist, 2605:34–41, 2007.
- T. Worstall. You know all those resources we’re about to run out of? No, we aren’t, 2014.
- I. A. Crawford. Lunar resources: A review. Progress in Physical Geography, 39(2): 137–167, 2015.
- F. Close. Fears over factoids. Physics World, 20(8):16–17, 2007.
- L. R. Nittler, T. J. McCoy, P. E. Clark, M. E. Murphy, J. I. Trombka, and E. Jarosewich. Bulk element compositions of meteorites: A guide for interpreting remote-sensing geochemical measurements of planets and asteroids. Antarctic Meteorite Research, 17:231–251, 2004.
- M. Elvis. Let’s mine asteroids — for science and profit. Nature, 485(7400):549–549, 2012
- K. Watson, B. Murray, and H. Brown. On the possible presence of ice in the Moon. Journal of Geophysical Research, 66(5):1598–1600, 1961
- B. A. Campbell, D. B. Campbell, J. F. Chandler, A. A. Hine, M. C. Nolan, and P. J. Perillat. Radar imaging of the lunar poles. Nature, 426(6963):137–138, 2003
- N. L. Chabot, C. M. Ernst, B. W. Denevi, H. Nair, A. N. Deutsch, D. T. Blewett, S. L. Murchie, G. A. Neumann, E. Mazarico, D. A. Paige, J. K. Harmon, J. W. Head, and S. C. Solomon. Images of surface volatiles in Mercury’s polar craters acquired by the MESSENGER spacecraft. Geology, 42(12):1051–1054, 2014.
- T. Platz, A. Nathues, N. Schorghofer, F. Preusker, E. Mazarico, S. E. Schröder, S. Byrne, T. Kneissl, N. Schmedemann, J.-P. Combe, M. Schäfer, G. S. Thangjam, M. Hoffmann, P. Gutierrez-Marques, M. E. Landis, J. Ripken, K. D. Matz, and C. T. Russel. Surface water-ice deposits in the northern shadowed regions of Ceres. Nature Astronomy, 1(7), 2016
- J. Haruyama, M. Ohtake, T. Matsunaga, T. Morota, C. Honda, Y. Yokota, C. M. Pieters, S. Hara, K. Hioki, K. Saiki, H. Miyamoto, A. Iwasaki, M. Abe, Y. Ogawa, H. Takeda, M. Shirao, A. Yamaji, and J.-L. Josset. Lack of exposed ice inside lunar south pole Shackleton Crater. Science, 322(5903):938–939, 2008
- A. Colaprete, P. Schultz, J. Heldmann, D. Wooden, M. Shirley, K. Ennico, B. Hermalyn, W. Marshall, A. Ricco, R. C. Elphic, D. Goldstein, D. Summy, G. D. Bart, E. Asphaug, D. Korycansky, D. Landis, and L. Sollitt. Detection of water in the LCROSS ejecta plume. Science, 330(6003):463–468, 2010.
- D. A. Paige, M. A. Siegler, J. A. Zhang, P. O. Hayne, E. J. Foote, K. A. Bennett, A. R. Vasavada, B. T. Greenhagen, J. T. Schofield, D. J. McCleese, M. C. Foote, E. DeJong, B. G. Bills, W. Hartford, B. C. Murray, C. C. Allen, K. Snook, L. A. Soderblom, S. Calcutt, F. W. Taylor, N. E. Bowles, J. L. Bandfield, R. Elphic, R. Ghent, T. D. Glotch, M. B. Wyatt, and P. G. Lucey. Diviner lunar radiometer observations of cold traps in the moon’s south polar region. Science, 330(6003): 479–482, 2010.